Process of Beta Sheet Assembly in Prion Hydrophobic Core
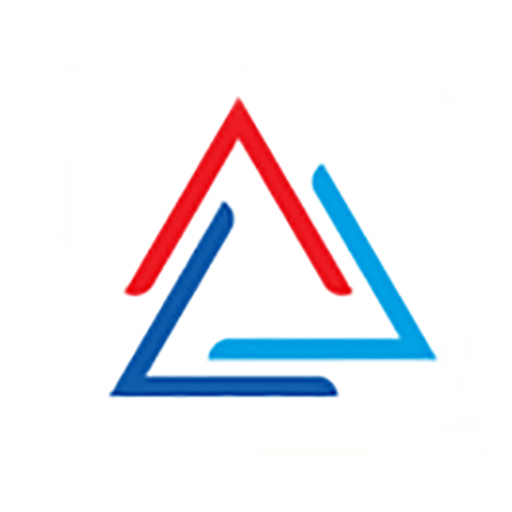
About the Research Project
Program
Award Type
Standard
Award Amount
$199,940
Active Dates
April 01, 1998 - March 31, 2000
Grant ID
A1998004
Goals
Process of Beta Sheet Assembly in Prion Hydrophobic Core
Summary
One of the hallmark pathological abnormalities of Alzheimer’s disease (AD) is the deposition of amyloid fibers in neuritic or senile plaques and in the walls of cerebral blood vessels. The major protein of AD amyloid, called Aß, derives from a much larger precursor protein, and can become toxic to nerve cells depending on the folding of the protein (conformation) and its aggregational state. Thus, the formation and accumulation of Aß amyloid correlates with the progressive devastation of memory, language and behavior occuring over the course of five to ten years or more. A somewhat similar mechanism is thought to underlie the pathophysiology of prion diseases, which however are considerably more acute, progressing in humans over a matter of months from first symptoms of death. These diseases, of which “mad-cow” disease is the most notorious, exist in heritable, infectious, and sporadic forms and in humans include Creuzfeldt-Jacob disease, Gerstmann-Straussler-Scheinker syndrome, fatal familial insomnia, and kuru. Similar molecular mechanisms involving the abnormal folding of protein are hypothesized to underlie both diseases. In the case of Alzheimer’s disease, a 40-or 42-amino acid long polypeptide fragment of the precursor protein, which likely exists as part of an a-helical domain in a membrane, folds into a ß-pleated sheet structure which then is induced to aggregate or intrinsically self-assembles into macromolecular assemblies called amyloid fibers. In the case of prion diseases, while there is also a conformational change from a predominantly a-helical cellular form of the prion protein to a ß-sheet form, the latter may or may not accumulate as macroscopic assemblies. In either case, what stabilizes the abnormal structures is considerable bonding between hydrogen atoms of the protein backbone and ionic and hydrophobic interactions between the sidechains of the proteins in the ß-sheet conformation. We plan to use x-ray diffraction and electron microscopy to characterize the details of this a helical ß-sheet transition in a model system that consists of synthetic protein fragments related to the prion protein. Correlation of results from these techniques will help to link the folding of the protein at the molecular level to images of its macromolecular aggregates. Knowing the three-dimensional organization of these stable ß-sheet structures will help us to understand how the initial genesis of the abnormal protein is promoted by point mutations, natural polymorphism, and physical-chemical factors; how interaction between the normal and abnormal proteins leads to the a->ß conversion; and how assemblies of the abnormally-folded protein is neurotoxic. Thus, this study will contribute to establishing the structural basis for prion pathophysiology. Our specific aims will address three hypotheses: The first is that the a->ß transition of the core region of the prion protein proceeds by intermolecular hydrogen-bonding of extended ß-chains followed by intra- and intermolecular ß-sheet stacking. The second is that point mutations in a specific domain of the protein and a naturally occurring variation in a different domain of the protein act together to modulate the folding process. The third hypothesis is that the neurotoxic region of the prion protein will self-assemble into a channel when exposed to the lipids that are found in neural membranes. Such a channel could allow a cytotoxic flow of ions. To undertake our research, we will use x-ray scatter (from solutions, gels & fibers) and electron micros- copy to study the conformation and self-assembly of synthetic peptides corresponding to different domains in the core region of the prion protein. Samples will be examined as a function of time of protein in solution (during which folding and self-assembly occurs), hydration (which affects formation of hydrogen- bonds and intersheet interactions), ionic strength (which determines the extent of shielding of electrically- charged groups in the protein) and pH (which fixes the concentration of hydrogen ions). Analysis of the samples will uniquely complement other biophysical/ultrastructural methods by directly relating molecular structure and protein folding to morphology and by giving information about molecular dimensions, the dispositions of the reactive sidechains of amino acid building blocks of proteins, and intercrystallite/proto- filament interactions in assemblages that closely resemble those in the disease. Such data will be essential for the rational development of anti-amyloid therapeutics that are directed at prion diseases, Alzheimer’s disease, and the other amyloid diseases.
Grants
Related Grants
Alzheimer's Disease Research
The Role of DYRK1A in Altered Microglia Biology in a Cellular Model of Alzheimer’s Disease in Down Syndrome
Active Dates
January 01, 2025 - December 31, 2027
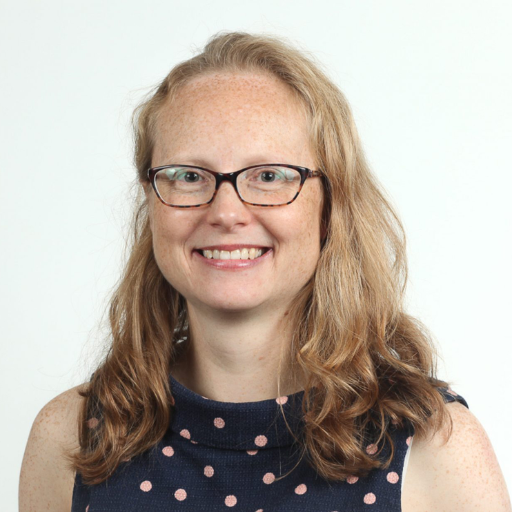
Principal Investigator
Frances Wiseman, PhD
Current Organization
University College London (UK)
Alzheimer's Disease Research
Decoding the Role of an Alzheimer's Causal Gene in Distinct Brain Cell Types
Active Dates
July 01, 2024 - June 30, 2027
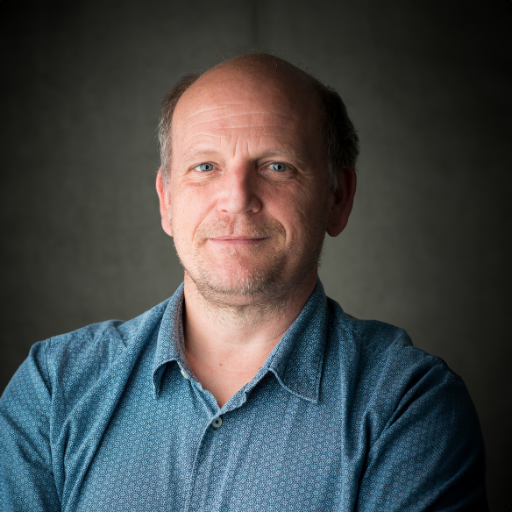
Principal Investigator
Willem Annaert, PhD
Current Organization
Flemish Institute for Biotechnology
Alzheimer's Disease Research
Staging Alzheimer's Disease Using Blood Samples
Active Dates
July 01, 2024 - June 30, 2026
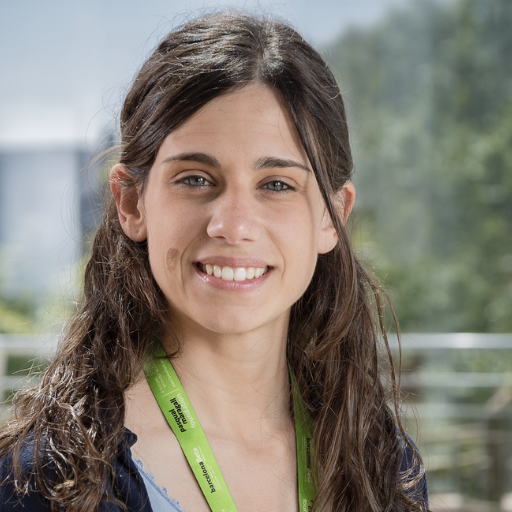
Principal Investigator
Gemma Salvadó, PhD
Current Organization
Lund University